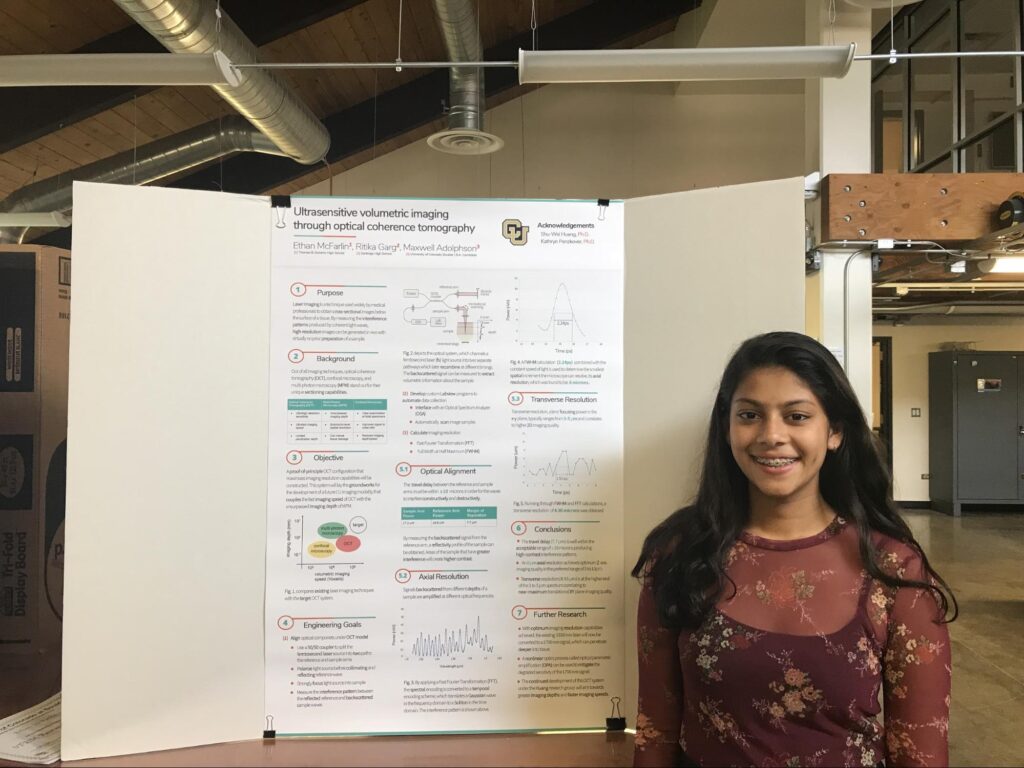
During the summer of 2018, in addition to the Molecular Neuroscience Course I took at the Rosetta Institute of Biomedical Research, I also took part in the STEM Research Experience at the University of Colorado at Boulder. As part of this program, I was given the privilege to work under the direct supervision of the PI of one of the lab’s at CU Boulder. I worked in the Huang Lab, under Dr. Shu-Wei Huang whose lab specialized in optics, nanostructures, and bioengineering. In Part 1 of my experiences at CU Boulder’s STEM Research Experience program, I will detail some of my personal experiences working in the Huang Lab as well as the main objective and premise of my research project.
From the very first day I went into the lab, I was absolutely captivated. The Huang lab was the very first professional lab I had the opportunity and pleasure of working in. I had my own desk where I could spend hours reading research papers or developing programs for my research project. I had my own station in the lab where I could fine tune every part of my project. I was in awe and I could not wait to start working.
As Dr. Huang made me aware, the project I would be working on was performing ultrasensitive volumetric imaging through optical coherence tomography. Now, those are a lot of big words, and I’m sure you’re wondering what they mean. Well, essentially, I would be working on creating a laser imaging system, known as optical coherence tomography (OCT), that would eventually be used to obtain cross-sectional images below the surface of a tissue, without ever actually making a single cut or incision in the tissue itself. The whole process would be completely non-invasive to the individual undergoing this process. Ultimately, the goal was to be able to image animal brains, such as fish or mice, and even human brains, if the technology was good enough.
Laser imaging is a technique widely used by medical professionals to obtain cross-sectional images of a tissue. By measuring the interference patterns produced by coherent light waves, high-resolution images can be generated in vivo with virtually no prior preparation of the sample.
Now, you may be wondering why I decided to pursue OCT, rather than other laser imaging techniques. Well, I considered three imaging techniques that stand out for their unique sectioning capabilities: optical coherence tomography (OCT), confocal microscopy, and multi-photon microscopy (MPM). Here is a table comparing the three imaging techniques:
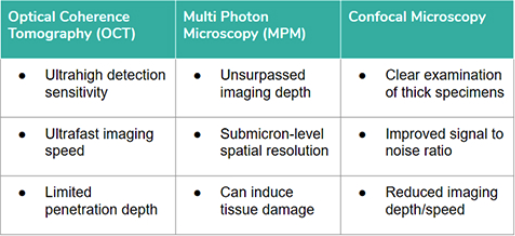
Ultimately, I chose OCT because of its superior qualities in relation to the other two imaging techniques.
The objective of my project was that an OCT configuration that maximizes imaging resolution capabilities will be constructed. This system would lay the groundwork for the development of a future CU Boulder imaging modality that combines the fast imaging of OCT with the unsurpassed imaging depth with MPM.
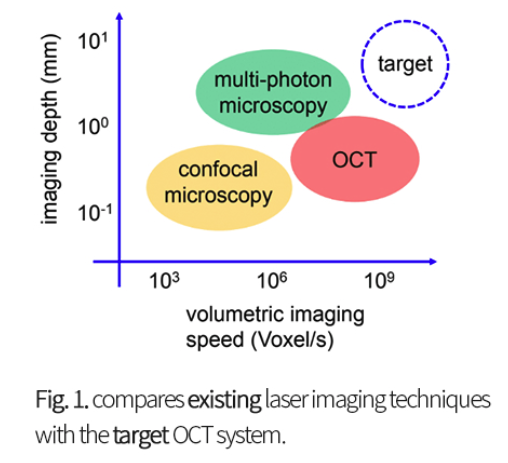
For the research project, I had several engineering goals: one, align optical components under the OCT model; two, develop custom Labview programs to automate data collections; three, calculate imaging resolution. To align the optical components under the OCT model, I had to use a 50/50 coupler to split the femtosecond laser (this is the specific name of the laser we used) into two paths: the reference arm and the sample arm. I also had to polarize the light source before collimating and reflecting the reference wave, strongly focus the laser into the sample, and measure the interference path between the reflected reference and the backscattered sample waves. This system is visually explained through Figure 2 below. To develop the custom Labview programs to automate data collections, I had to figure out how to interface the program with an Optical Spectrum Analyzer (OSA), on which I would be collecting my data, and automatically scan image samples. Finally to calculate the imaging resolution, I, with the help of my Dr. Huang, had to calculate the Fast Fourier Transformation (FFT) and the Full Width at Half Maximum (FWHM).
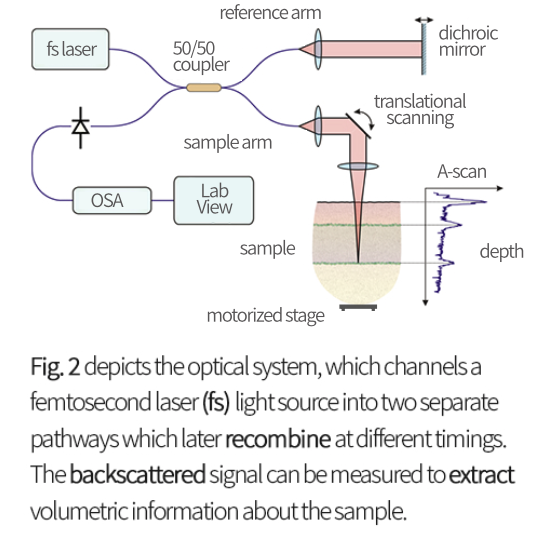
This is what the configuration looked like in real life, while I was working on it:
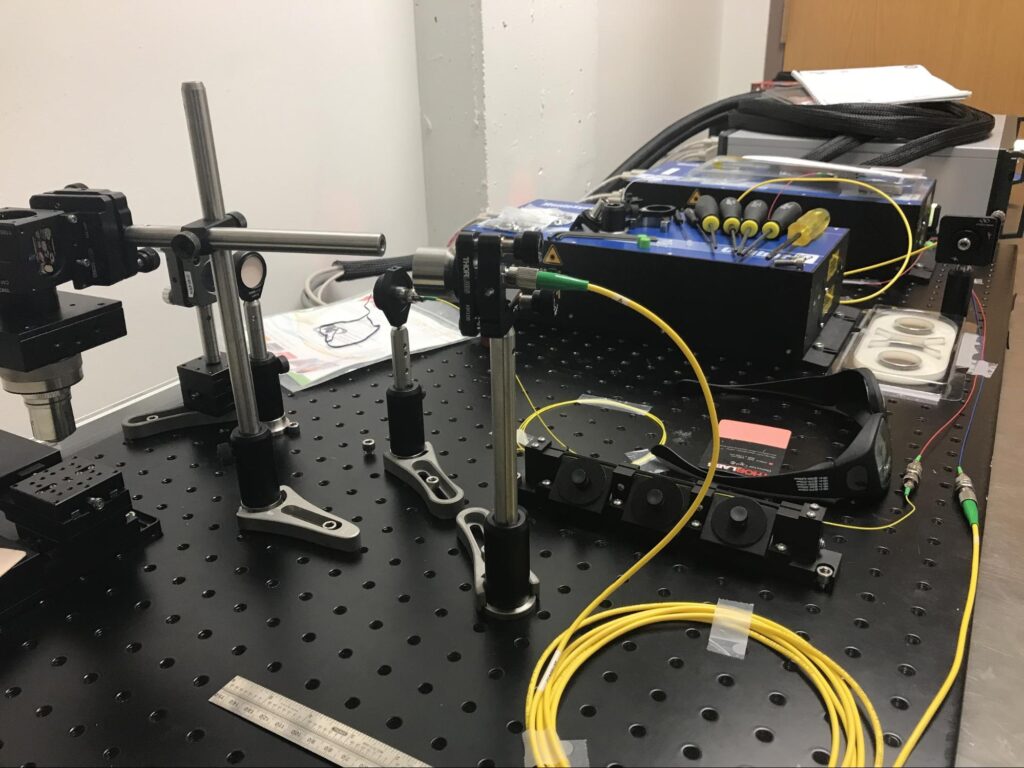
For the first week or so that I was at the STEM Research Experience, I sat at my station in the lab for hours and performed the grueling task of configuring the laser so that it formed the perfect configuration to create the desired frequency of light to perform optical coherence tomography (OCT). I would make a slight change to the laser, barely increasing the height of the podium or shifting the target left or right, just to realize that I overshot and I have to start all over again. Although it took me a week of continuous work to perfect the laser system, I had fun. I enjoyed learning about the mechanism of the laser. I enjoyed using a screwdriver to attach nails, podiums, and stands into the plastic board on which I was building my laser configuration. I enjoyed frantically searching the lab for just the perfect height for the podium so that my laser would hit the exact center of the reflective mirror or prism directly opposite to it. Again and again, I would run to the back of the lab and switch off the light to see where the laser was hitting the target in front of it. I would hold up a laser detector card from Thor Labs to check and double check and triple check what the laser’s path was. It was hard work — there’s no doubt about that. But, I had fun and I learned so much because I was able to devote time from 9am to 5pm in the lab every single day for four weeks.
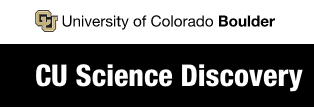