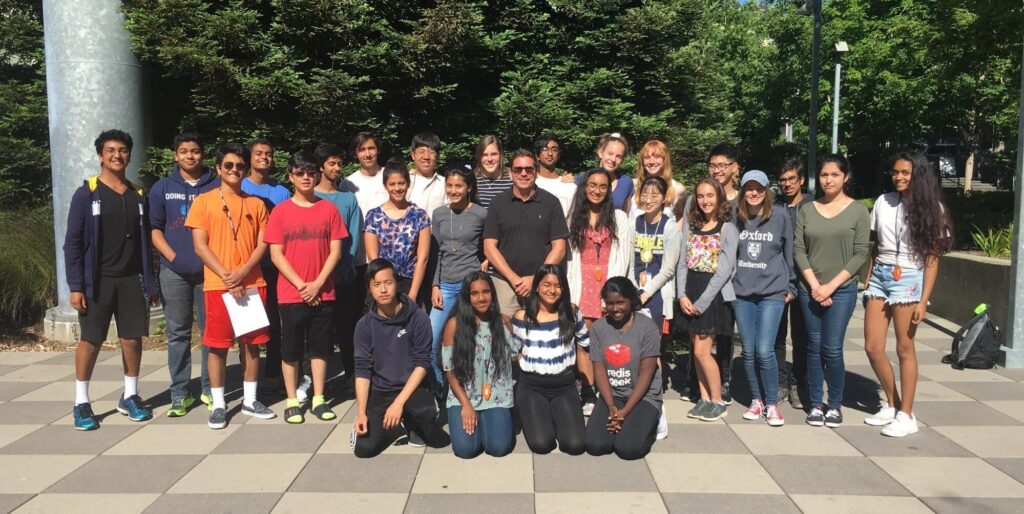
From June 7th to June 20th, I was at UC Berkeley for the Rosetta Institute of Biomedical Research Molecular Neuroscience summer camp. It was not only an exhilarating experience, but an extremely educational one. I was able to learn so much about one of the body’s most fascinating organs in such little time with the help of a great professor, Dr. Troy Rohn from Boise State University, and several team advisors. I loved Dr. Rohn’s teaching style because not only did he give great lectures (reference Post 1 for my favorite learnings from his course), but also he used case studies that taught me the same basics of neuroscience in a different way and taught me to apply what I had learned. This post is the second of a two-part series covering the highlights of my experience.
Case Studies
First of all, what are case studies? Case studies are a research method that involve a detailed investigation of the subject of the study. Case studies help in understanding the complexity of an issue or object. They can either advance the knowledge of a topic in a particular field or they can strengthen existing knowledge of topics in a field. Usually, case studies track individuals whose cases were harder for researchers and doctors to initially diagnose. In this situation, case studies can be extremely important learning opportunities as they allow future researchers and doctors to learn unique or novel presentations of the symptoms of certain diseases.
In my course at Rosetta, Dr. Rohn strongly believed in learning from case studies. He believed that giving real life applications to the molecular neuroscience topics we were learning was a great way to have fun with science as well as learn things that we would not otherwise get to.
Case Study 1: Phineas Gage
In 1848, a 25-year-old railroad worker named Phineas Gage was blowing up rocks to clear the way for a new rail line in Cavendish, Vermont. He would drill a hole, place an explosive charge, then pack in sand using a metal bar known as a tamping iron. But, in this instance, the metal bar created a spark that touched the charge. That, in turn, drove this 13-pound tamping iron up and out of the hole, through his left cheek, behind his eye socket, and out the top of his head.
Surprisingly, though, Gage did not die. However, the tamping iron destroyed much of his brain’s left frontal lobe, and Gage’s once even-tempered personality changed dramatically. “He is fitful, irreverent, indulging at times in the grossest profanity, which was not previously his custom,” wrote John Martyn Harlow, the physician who treated Gage after his accident.
Gage’s sudden personality change was the reason he shows up in so many medical textbooks; he was the first case where scientists could definitely say that injury to the brain produced some kind of change in personality. This conclusion was a huge deal in the mid-1800s when the brain’s purpose and inner workings were largely a mystery. Gage’s famous case would ultimately help establish “brain science” as a field. Phineas Gage’s case was ideal because the damage was located in only one region of the brain and it was the starting point for scientists’ investigation into the relationship between structural damage to the brain and particular changes in behavior.
Following Gage’s case, generations of neuroscientists have revisited Gage’s case, each one making different discoveries: in the 1940s, Stanley Cobb (a renowned neurologist) diagrammed the skull to determine the exact path the tamping iron took; in the 1980s, scientists repeated the exercise from 1940 but they used CT scans; in the 1990s, researchers applied 3D computer modeling to the problem; in 2012, a team combined CT scans of Gage’s brain with MRI scans of normal brains to show how the wiring of Gage’s brain could have been affected.
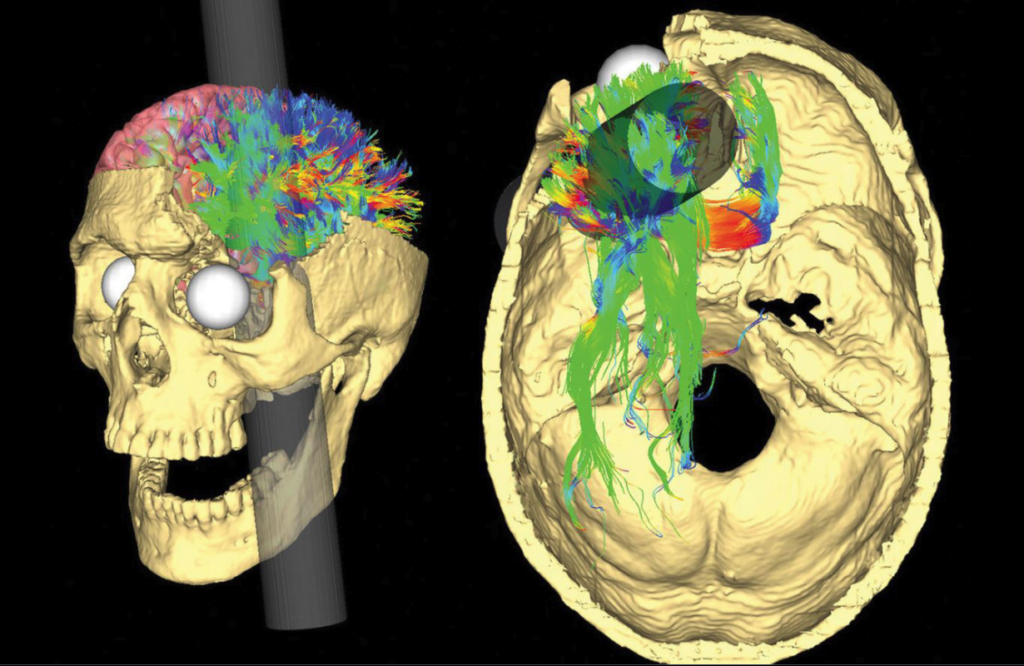
Even though Gage’s case may seem outdated, doctors still apply it to this day when assessing patients. Every year, neuroscientists and neurologists are faced with cases of patients that have been shot in the head with an arrow or have fallen off a ladder and landed on a piece of metal, all of which are modern Phineas Gage-like cases.
Another key application of the Phineas Gage case was his remarkable recovery to normalcy. Many people do not know that Gage’s personality change lasted about two or three years, after which he went on to work as a long-distance stagecoach driver in Chile, a job that requires considerable planning skills and focus. This chapter of Gage’s life offers a powerful message about brain plasticity, which is the brain’s ability to adapt as a result of experience or change, as well as brain rehabilitation.
Ultimately, Phineas Gage lived for a dozen years after his accident. However, the brain damage he had sustained probably contributed to his death as he died on May 21, 1860 of an epileptic seizure. Now, Gage’s skull, and the tamping iron that ripped through it, are on display at the Warren Anatomical Museum in Boston, Massachusetts.
Case Study 2: Bad Fish, Bad Bird
One evening during a trip to Indonesia to study the recent sightings of a coelacanth, Dr. Marshall Westwood from the Montana Technical Institute ate a meal of pufferfish. Within hours of returning to his hotel room, he felt numbness in his lips and tongue, which quickly spread to his face and neck, and he began to feel pains in his stomach and throat, which produced feelings of nausea and eventually severe vomiting. Dr. Westwood called a local hospital. NUmbness in his face and lips made it almost impossible for him to communicate, but the hospital staff sent an ambulance. As Dr. Westwood was rushed to the hospital, he had trouble breathing as well as signs of paralysis in his upper body and arms. The physicians at the hospital kept his airway open, administered drugs to bring his heart back to normal rhythm, and put a mixture of charcoal into his stomach to absorb any chemicals that might still be there. Within a few hours, Dr. Westwood’s condition improved and he was on his way to a full recovery.
So, what could have caused Dr. Westwood to experience such severe symptoms? If you guessed the meal of pufferfish he ate that evening, you are correct! After discussing his case with his physician, Dr. Westwood learned that he had probably been a victim of pufferfish poisoning.
Now, ask yourself: what could the pufferfish meal have contained that was potent enough to almost kill Dr. Westwood?
If you guessed that Dr. Westwood had been the victim of pufferfish poisoning, you guessed correctly! The active toxin in the tissues of the pufferfish is a chemical called tetrodotoxin (TTX), a neurotoxin that affects nerve cells, or neurons. TTX blocks voltage-gated sodium ion channels. The image below shows a voltage-gated sodium ion channel. Voltage gated sodium ion channels are integral membrane proteins that change their spatial arrangement in response to depolarization of the membrane potential, open a pore that goes from the outside the cell to inside the cell, and conduct sodium ions inward to initiate and propagate action potentials.
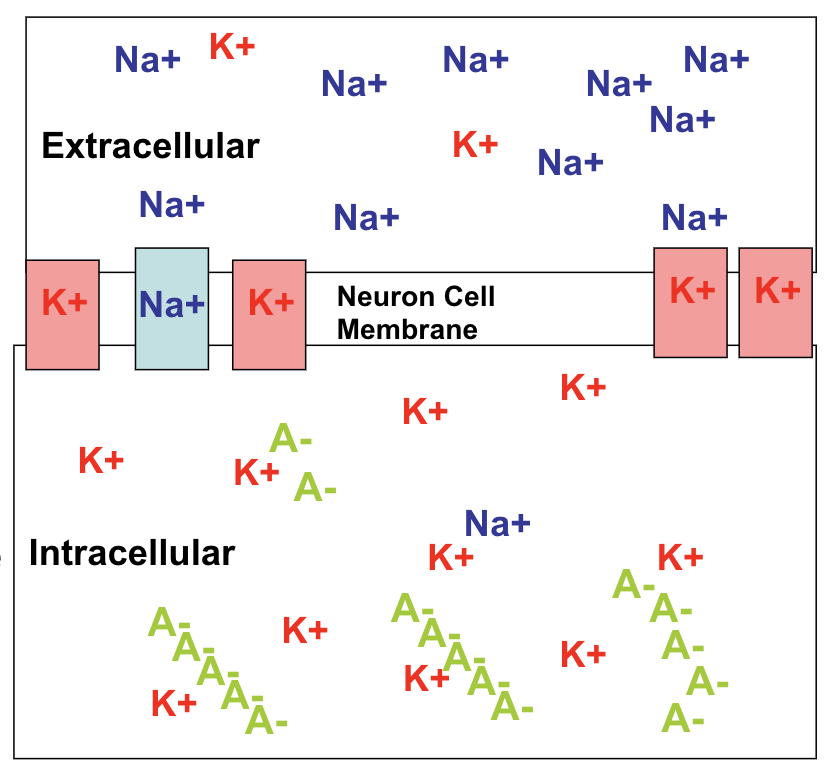
TTX blocks the process described above: when TTX binds to the sodium ion channels, it effectively prevents sodium ions from entering the channel. This would prevent the cell from depolarizing and block the cell from generating an action potential. Since the action potential is the signal that neurons use in cell-to-cell communication, the ability of a neuron to communicate would be inhibited.
Now, you may be asking: what does all this information have to do with the fact that Dr. Westwood experienced paralysis? Well, muscles contract after receiving signals from motor neurons. Since TTX prevents the generation of action potentials in these neurons, the ability of a motor neuron to generate this signal will be inhibited, resulting in an emergence of paralysis in the affected areas. Sodium ions also contribute to the action potential generated in muscle cells, so TTX inhibits muscle activity directly.
After reading all of this, you might also be wondering why species that have TTX are not susceptible to the effects of TTX? These species are not susceptible to the effects of TTX because the voltage-gated sodium channels, in species that have TTX, work differently than the voltage-gated sodium channels in humans.
Conclusion
In conclusion, case studies, like the two described above, were an integral part of my learning at the Molecular Neuroscience Course at the Rosetta Institute of Biomedical Research summer camp. Each case study presented the same neuroscience information as a regular lecture would, but instead the case studies engaged me and forced me to really consider what I had previously learned and apply it to the situation at hand.
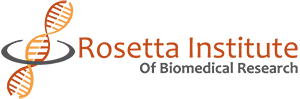